Meet Our Principal Investigator
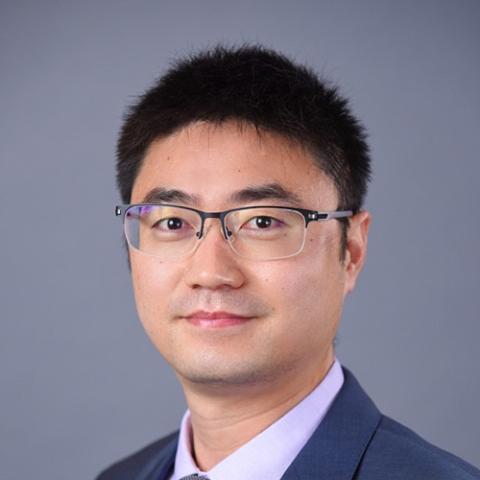
Nian Wang, Ph.D.
Principal Investigator
Associate Professor
Advanced Imaging Research Center
Department of Biomedical Engineering
Peter O'Donnell Jr. Brain Institute
Dr. Nian Wang received his B.S. from Wuhan University, and his Ph.D. degree at Chinese Academy of Sciences. He worked at Duke University as a research associate and then promoted to an assistant professor in 2018. Dr. Wang joined Indiana University School of Medicine as a tenure-track assistant professor in 2020. Dr. Wang’s interests include developing novel MRI acquisition and reconstruction methods for various brain diseases and musculoskeletal disorders.
Current Lab Members
Xinyue Han, Ph.D.
Research Associate
UT Southwestern Medical Center
Diffusion MRI, Multimodality Imaging Analysis
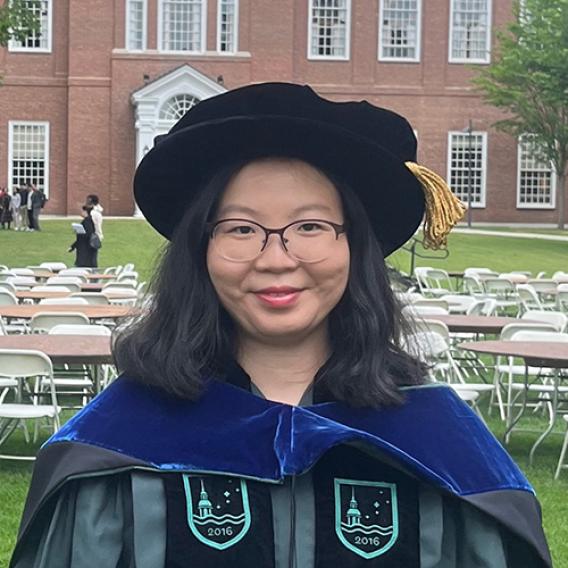
Jie Chen, Ph.D.
Research Associate
UT Southwestern Medical Center
Imaging Analysis, Deep Learning
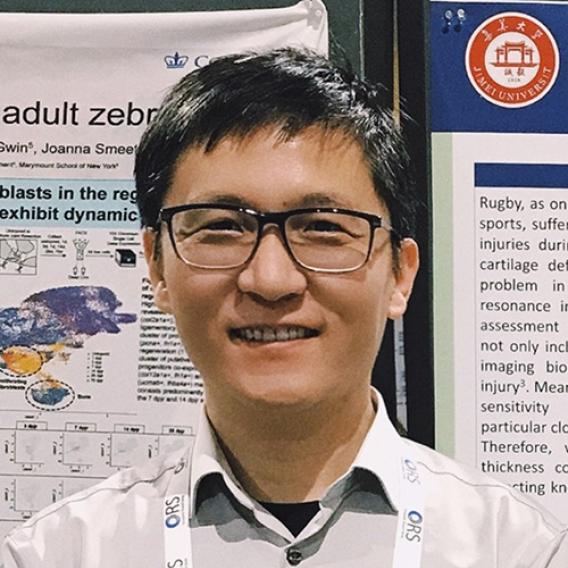
Zhuoheng Liu, M.S.
Graduate Student
UT Southwestern Medical Center
Deep Learning, Diffusion MRI
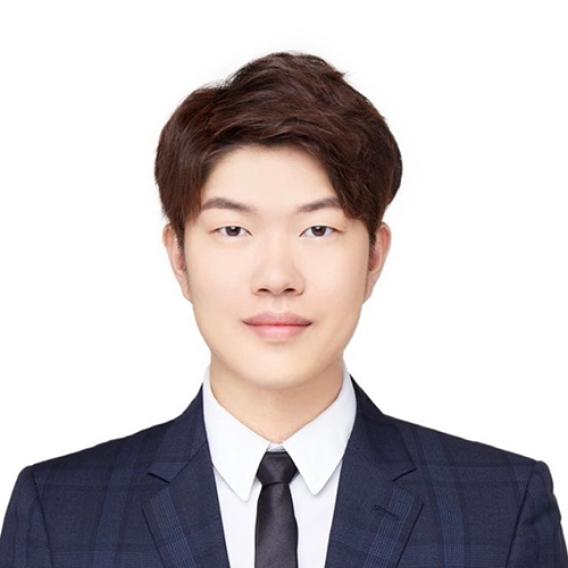
Saira Tabassam, M.S.
Research Assistant
UT Southwestern Medical Center
Cancer Biology, Neurology
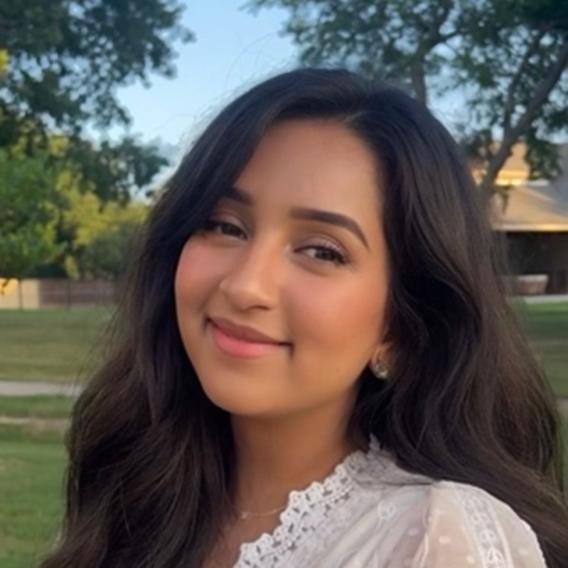
Rui Hu, M.S.
Visiting Student
UT Southwestern Medical Center
Medical Imaging Analysis, Deep Learning
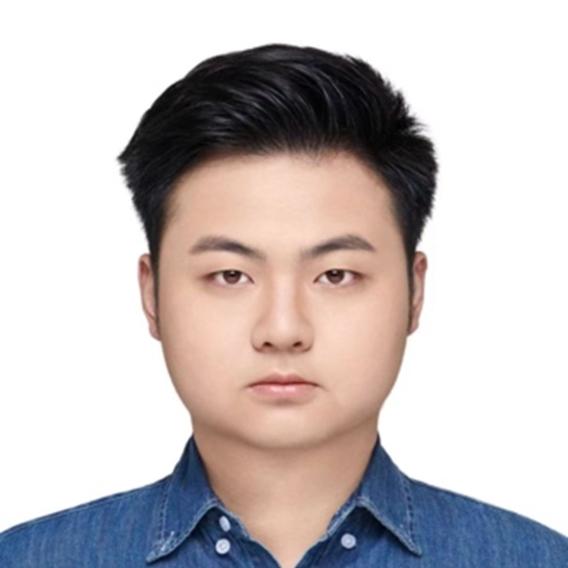
Nataliya Tod, B.S.
Perot Family MSTP Scholar
UT Southwestern Medical Center
Biochemistry, MSK Imaging
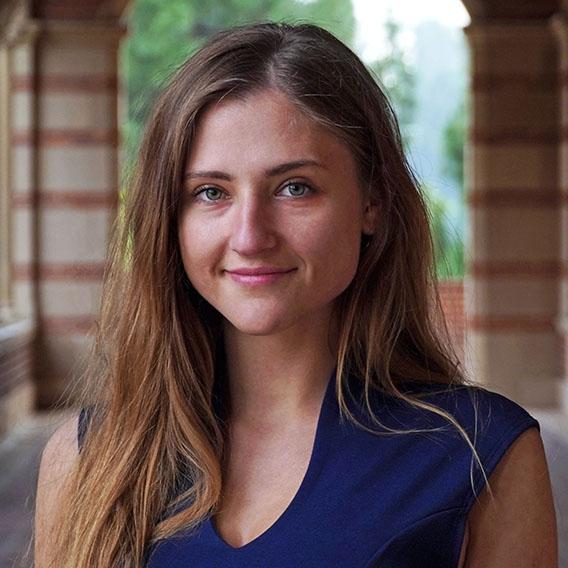
Hongbo Wu, M.S.
Research Assistant
UT Southwestern Medical Center
Neural Interface, Biosensor, Machine Learning
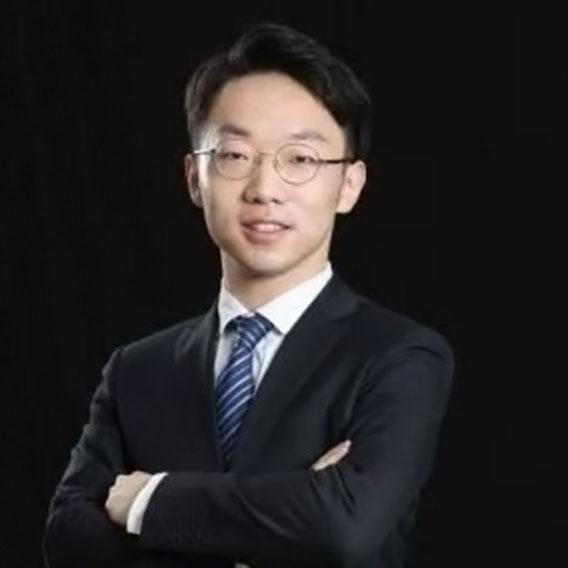
Summer Intern Students
- Mubashir Jafry
- Pratham Eddula
- Ishaan Mehta
Research
The Laboratory of Nian Wang, Ph.D., is focused on developing novel quantitative MRI techniques and analysis methods on CNS and musculoskeletal system. The research group has developed advanced MRI techniques to detect the early change of Multiple Sclerosis, Alzheimer’s disease, and ASD, including UTE, multi-component T2*, quantitative susceptibility mapping (QSM) and compressed sensing for high-resolution diffusion MRI.
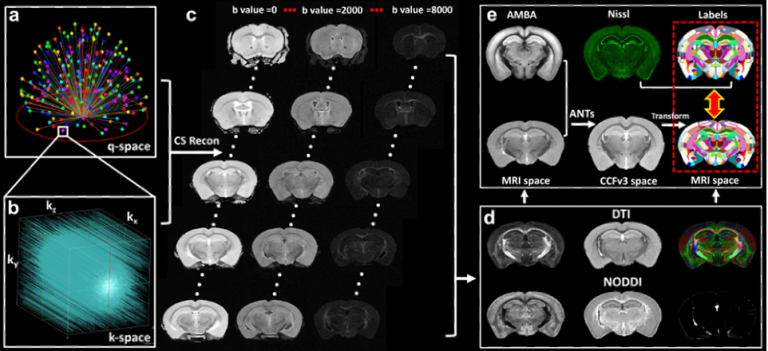
High-resolution MRI affords unique image contrasts to non-destructively probe the tissue microstructure. To better validate various MRI metrics, we acquired whole mouse brain high-resolution quantitative susceptibility mapping (QSM) and diffusion MRI, and registered to the Allen Mouse Brain Common Coordinate Framework (CCFv3). Integrating different imaging modalities to the same space may substantially improve our understanding of the complexity of the brain at different scales.
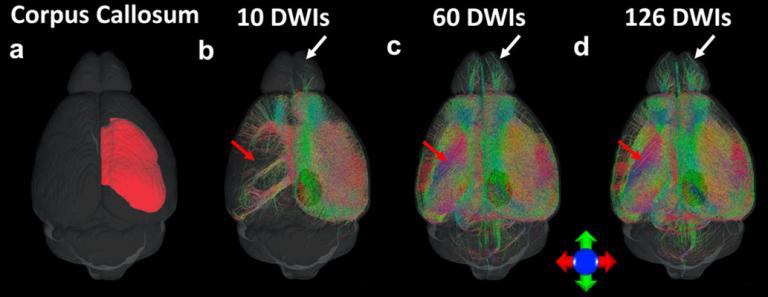
Diffusion magnetic resonance imaging has been widely used in both clinical and preclinical studies to characterize tissue microstructure and structural connectivity. The diffusion MRI protocol for the Human Connectome Project (HCP) has been developed and optimized to obtain high-quality, high-resolution diffusion MRI (dMRI) datasets. However, such efforts have not been fully explored in preclinical studies, especially for rodents. In this study, high quality dMRI datasets of mouse brains were acquired at 9.4T system from two vendors. Our study provides guidelines and foundations for exploration of tradeoffs among acquisition parameters for the structural connectome in ex vivo mouse brain.
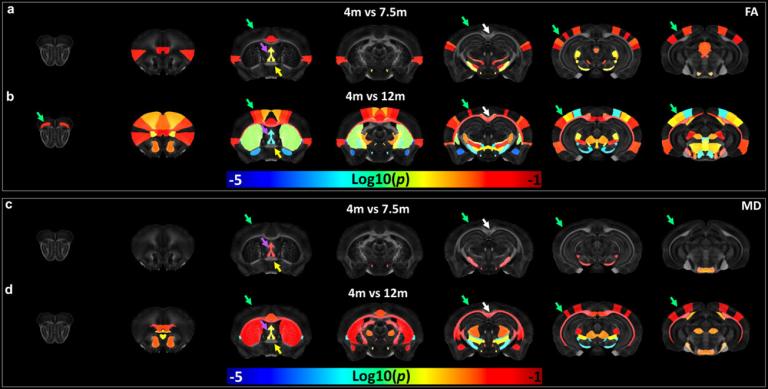
Alzheimer’s disease (AD) is the most common cause of dementia with clinically characterized by age-dependent memory loss and cognitive dysfunction. The capability to monitor the alterations in tissue microstructure caused by the accumulation of Aβ may provide an important biomarker to monitor disease progression or treatment response. We have evaluated the age-dependent microstructure changes in 5xFAD mice using high-resolution diffusion tensor imaging (DTI). FA showed higher sensitivity to monitor amyloid deposition in 5xFAD mice. DTI may be utilized as a sensitive biomarker to monitor beta-amyloid progression for preclinical studies.
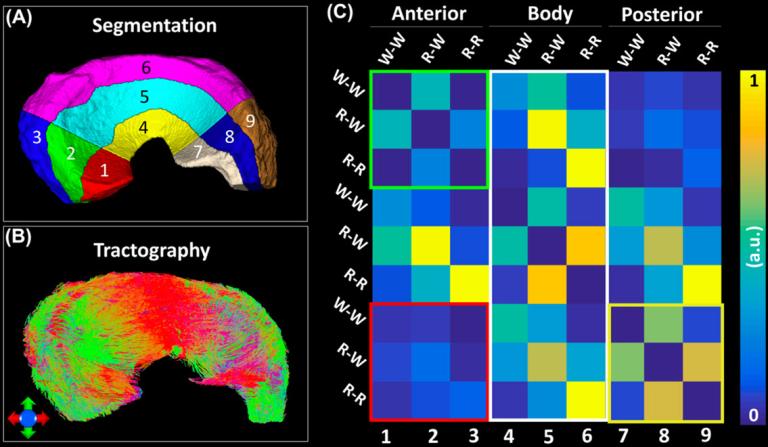
Conventional morphological MRI relies on the assessment of surface integrity and sub-surface signal intensity as indicators of tissue defects, which is qualitative and has limitations to detect the meniscal composition changes before surface breakdown or small tears. dMRI and tractography in musculoskeletal system has attracted more and more attention to investigate the tissue microstructure, local collagen fiber alignment, and the 3D collagen network. To noninvasively evaluate the three-dimensional collagen fiber architecture of porcine meniscus using diffusion MRI, meniscal specimens were scanned using a 3D diffusion-weighted spin-echo pulse sequence at 7.0 T. The tractography based on diffusion MRI may provide a complementary method to study the integrity of meniscus and nondestructively visualize the 3D collagen fiber architecture.
Featured Publications
- Han X.Y., et al., High-resolution Diffusion Magnetic Resonance Imaging and Spatial-Transcriptomic in Developing Mouse Brain. NeuroImage, 2024. 297(15): 120734.
- Kotredes K.P., et al., Characterizing molecular and synaptic signatures in mouse models of late‐onset Alzheimer's disease independent of amyloid and tau pathology. Alzheimer's & Dementia, 2024. In Press.
- Zhao Q. et al., High angular resolution diffusion imaging (HARDI) of porcine menisci: a comparison of diffusion tensor imaging and generalized q-sampling imaging. Quantitative Imaging in Medicine and Surgery, 2024. 14(4): 2738-2746.
- Raya, J.G., et al., Applications of Diffusion-Weighted MRI to the Musculoskeletal System. J Magn Reson Imaging, 2024. 59(2): p. 376-396.
- Wang, N., et al., Integrating multimodality magnetic resonance imaging to the Allen Mouse Brain Common Coordinate Framework. NMR in Biomedicine, 2023. 36(5).
- Tsai, A.P., et al., Genetic variants of phospholipase C-gamma2 alter the phenotype and function of microglia and confer differential risk for Alzheimer's disease. Immunity, 2023. 56(9): p. 2121-2136 e6.
- Johnson, G.A., et al., Merged magnetic resonance and light sheet microscopy of the whole mouse brain. Proc Natl Acad Sci U S A, 2023. 120(17): p. e2218617120.
- Jang, A., et al., B(1) inhomogeneity-corrected T(1) mapping and quantitative magnetization transfer imaging via simultaneously estimating Bloch-Siegert shift and magnetization transfer effects. Magn Reson Med, 2023. 90(5): p. 1859-1873.
- Wang, N., et al., Magic angle effect on diffusion tensor imaging in ligament and brain. Magn Reson Imaging, 2022. 92: p. 243-250.
- Shen, J., et al., Tractography of Porcine Meniscus Microstructure Using High-Resolution Diffusion Magnetic Resonance Imaging. Front Endocrinol (Lausanne), 2022. 13: p. 876784.
- Maharjan, S., et al., Age-dependent microstructure alterations in 5xFAD mice by high-resolution diffusion tensor imaging. Front Neurosci, 2022. 16: p. 964654.
- Jackson, L.R., et al., Use of multimodality imaging, histology, and treatment feasibility to characterize a transgenic Rag2-null rat model of glioblastoma. Front Oncol, 2022. 12: p. 939260.
- Crater, S., et al., Resolution and b value dependent structural connectome in ex vivo mouse brain. Neuroimage, 2022. 255: p. 119199.
- Zhao, Q., et al., Effects of Angular Resolution and b Value on Diffusion Tensor Imaging in Knee Joint. Cartilage, 2021. 13(2_suppl): p. 295S-303S.
- Garrett, A., et al., Mapping the peripheral nervous system in the whole mouse via compressed sensing tractography. J Neural Eng, 2021. 18(4).
- Wang, N., et al., Cytoarchitecture of the mouse brain by high resolution diffusion magnetic resonance imaging. Neuroimage, 2020. 216.
- Wang, N., et al., Characterization complex collagen fiber architecture in knee joint using high-resolution diffusion imaging. Magn Reson Med, 2020.
- Wang, N., F. Badar, and Y. Xia, Resolution-dependent influences of compressed sensing in quantitative T2 mapping of articular cartilage. Nmr in Biomedicine, 2020. 33(12).
- Wang, N., et al., Variability and heritability of mouse brain structure: Microscopic MRI atlases and connectomes for diverse strains. Neuroimage, 2020. 222.
- Wang, N., et al., Probing demyelination and remyelination of the cuprizone mouse model using multimodality MRI. Journal of Magnetic Resonance Imaging, 2019. 50(6): p. 1852-1865.
- Wang, N., et al., Neurite orientation dispersion and density imaging of mouse brain microstructure. Brain Struct Funct, 2019. 224(5): p. 1797-1813.
- Wang, N., et al., Diffusion tractography of the rat knee at microscopic resolution. Magn Reson Med, 2019. 81(6): p. 3775-3786.
- Privratsky, J.R., et al., Dynamic contrast-enhanced MRI promotes early detection of toxin-induced acute kidney injury. Am J Physiol Renal Physiol, 2019. 316(2): p. F351-F359.
- Allan Johnson, G., et al., Whole mouse brain connectomics. J Comp Neurol, 2019. 527(13): p. 2146-2157.
- Wang, N., et al., Accelerating quantitative susceptibility imaging acquisition using compressed sensing. Phys Med Biol, 2018. 63(24): p. 245002.
- Wang, N., F. Badar, and Y. Xia, Compressed sensing in quantitative determination of GAG concentration in cartilage by microscopic MRI. Magnetic Resonance in Medicine, 2018. 79(6): p. 3163-3171.
- Wang, N., et al., Whole mouse brain structural connectomics using magnetic resonance histology. Brain Struct Funct, 2018. 223(9): p. 4323-4335.
- Wei, H., et al., Joint 2D and 3D phase processing for quantitative susceptibility mapping: application to 2D echo-planar imaging. NMR Biomed, 2017. 30(4).
- Wei, H., et al., Susceptibility tensor imaging and tractography of collagen fibrils in the articular cartilage. Magn Reson Med, 2017. 78(5): p. 1683-1690.
- Wei, H., et al., Investigating magnetic susceptibility of human knee joint at 7 Tesla. Magn Reson Med, 2017. 78(5): p. 1933-1943.
- Guan, X., et al., Influence of regional iron on the motor impairments of Parkinson's disease: A quantitative susceptibility mapping study. J Magn Reson Imaging, 2017. 45(5): p. 1335-1342.
- Guan, X., et al., Regionally progressive accumulation of iron in Parkinson's disease as measured by quantitative susceptibility mapping. NMR Biomed, 2017. 30(4).
- Cronin, M.J., et al., Exploring the origins of echo-time-dependent quantitative susceptibility mapping (QSM) measurements in healthy tissue and cerebral microbleeds. Neuroimage, 2017. 149: p. 98-113.
- Xie, L., et al., Dynamic contrast-enhanced quantitative susceptibility mapping with ultrashort echo time MRI for evaluating renal function. Am J Physiol Renal Physiol, 2016. 310(2): p. F174-82.
- Wei, H., et al., Streaking artifact reduction for quantitative susceptibility mapping of sources with large dynamic range. NMR Biomed, 2015. 28(10): p. 1294-303.
- Wang, N., et al., Molecular origin of a loading-induced black layer in the deep region of articular cartilage at the magic angle. J Magn Reson Imaging, 2015. 41(5): p. 1281-90.
- Wang, N., F. Badar, and Y. Xia, MRI properties of a unique hypo-intense layer in degraded articular cartilage. Phys Med Biol, 2015. 60(22): p. 8709-21.
- Li, W., et al., A method for estimating and removing streaking artifacts in quantitative susceptibility mapping. Neuroimage, 2015. 108: p. 111-22.
- Wang, N. and Y. Xia, Anisotropic analysis of multi-component T2 and T1rho relaxations in Achilles tendon by NMR spectroscopy and microscopic MRI. J Magn Reson Imaging, 2013. 38(3): p. 625-33.
- Wang, N. and Y. Xia, Experimental issues in the measurement of multi-component relaxation times in articular cartilage by microscopic MRI. J Magn Reson, 2013. 235: p. 15-25.
- Wang, N., E. Chopin, and Y. Xia, The effects of mechanical loading and gadolinium concentration on the change of T1 and quantification of glycosaminoglycans in articular cartilage by microscopic MRI. Phys Med Biol, 2013. 58(13): p. 4535-47.
- Wang, N. and Y. Xia, Depth and orientational dependencies of MRI T(2) and T(1rho) sensitivities towards trypsin degradation and Gd-DTPA(2-) presence in articular cartilage at microscopic resolution. Magn Reson Imaging, 2012. 30(3): p. 361-70.
- Wang, N. and Y. Xia, Orientational dependent sensitivities of T2 and T1rho towards trypsin degradation and Gd-DTPA2- presence in bovine nasal cartilage. MAGMA, 2012. 25(4): p. 297-304.
- Xia, Y., et al., Strain-dependent T1 relaxation profiles in articular cartilage by MRI at microscopic resolutions. Magn Reson Med, 2011. 65(6): p. 1733-7.
-
Wang, N. and Y. Xia, Dependencies of multi-component T2 and T1rho relaxation on the anisotropy of collagen fibrils in bovine nasal cartilage. J Magn Reson, 2011. 212(1): p. 124-32.
Join Our Lab
Be part of the great impact we're having on science and medical care across the globe.
We are looking for self-motivated candidates who are passionate about brain structure and function and interested in Neuroimaging and Neurodegenerative diseases. Rotation, visiting, and Ph.D. students may have a research background in Neuroscience, Biomedical Engineering, Computer Science, Physics, or Mathematics. Postdoc applicants must have (or expect to receive) a Ph.D., M.D., or M.D./Ph.D. degree with prior experience in one of the following research areas: 1) MRI pulse sequence development, image reconstruction, or imaging data analysis; 2) neurodegenerative diseases, such as Alzheimer's disease and multiple Sclerosis; or 3) Machine learning/AI in medical imaging segmentation, registration, or analysis. To apply, please send a single email containing your CV, a short statement describing your qualifications for this position and research interests, as well as contact information for three references to Dr. Nian Wang.
Lab Fun
Science and Art
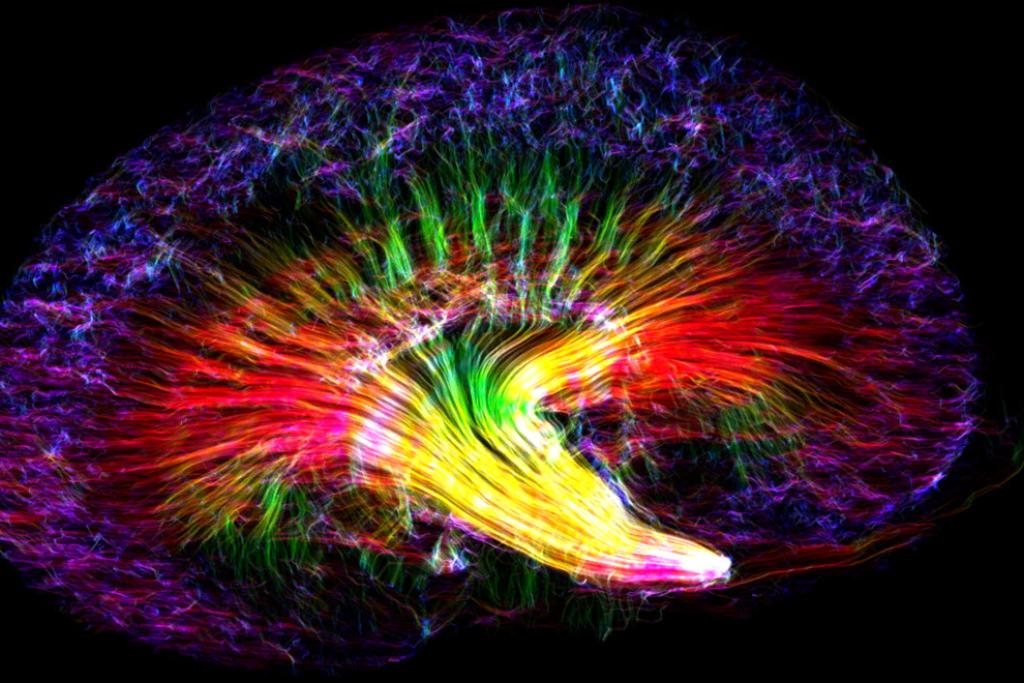
BMC "research in progress" winning image
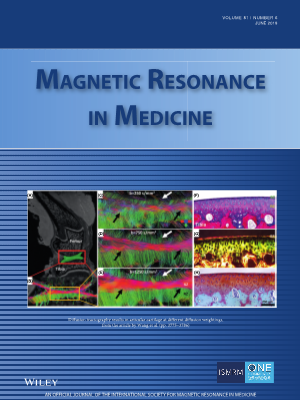
Cover Article in Magnetic Resonance in Medicine– Diffusion Tractography of Knee Cartilage
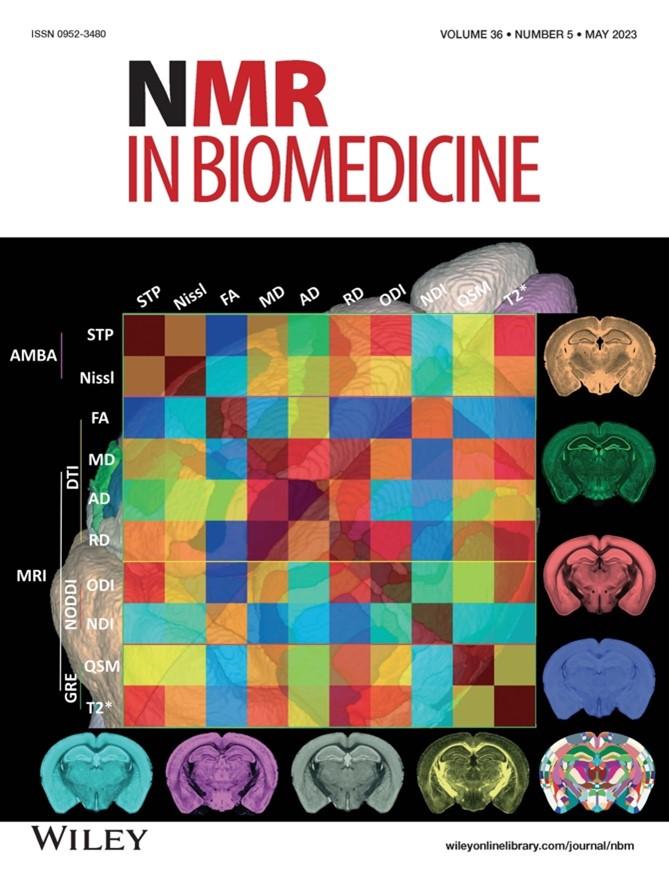
Cover Article in NMR in Biomedicine – Multimodality Imaging of Mouse Brain
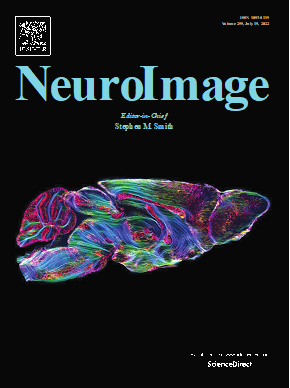
Cover Article in NeuroImage – High-resolution Track Density Imaging of Mouse Brain
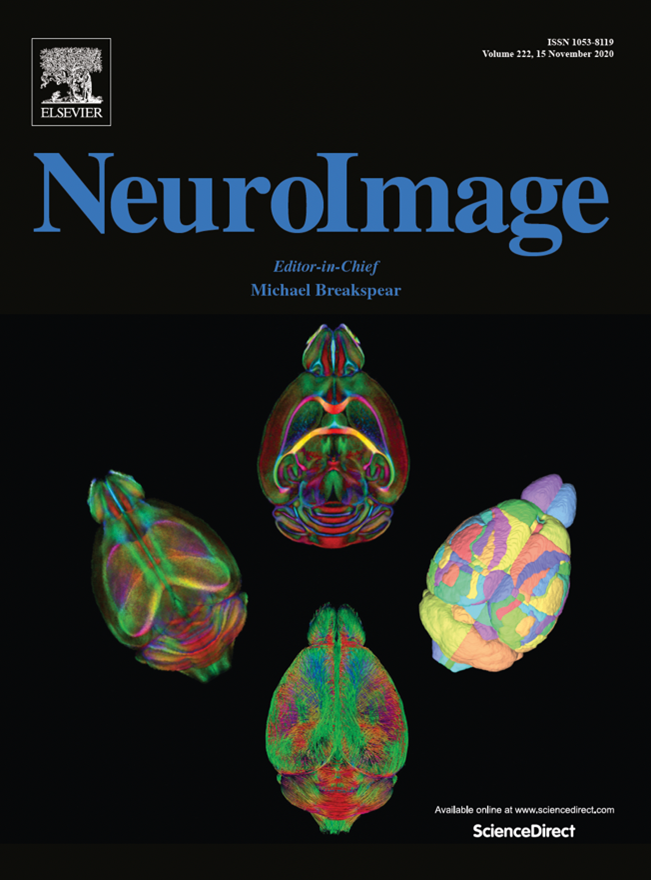
Cover Article in NeuroImage –Mouse Brain Atlas by diffusion MRI