1. Roles of membrane contact sites in intracellular lipid trafficking and homeostasis in response to cellular stress.
Membrane contact sites (MCSs) are regions where signals and small molecules, such as lipids, are exchanged between organelles. The Prinz lab studies the mechanism and function of lipid exchange at MCSs using biochemical and cell biological approaches. An important open question is how the structure and biophysical properties of MCSs modulate lipid exchange and how these properties are modulated in response to cellular stresses.
Lipid exchange at contact sites requires lipid transport proteins (LTPs), which shuttle lipids between membranes. Recently, a new family of tube-like LTPs has been proposed to use a different mechanism; they are thought to bind two membranes simultaneously and allow lipids to flow between the membranes. Notably, mutations in most of the human proteins in this LTP family cause or are associated with neurodegenerative and other diseases. Current work focuses on understanding the roles of the LTPs in facilitating lipid transport in cells and maintaining intracellular membrane fluidity with an eye toward gaining mechanistic insight into the roles of the LTPs in disease. We are also investigating the mechanism of lipid transport by tube-like LTPs in vitro using model membranes.
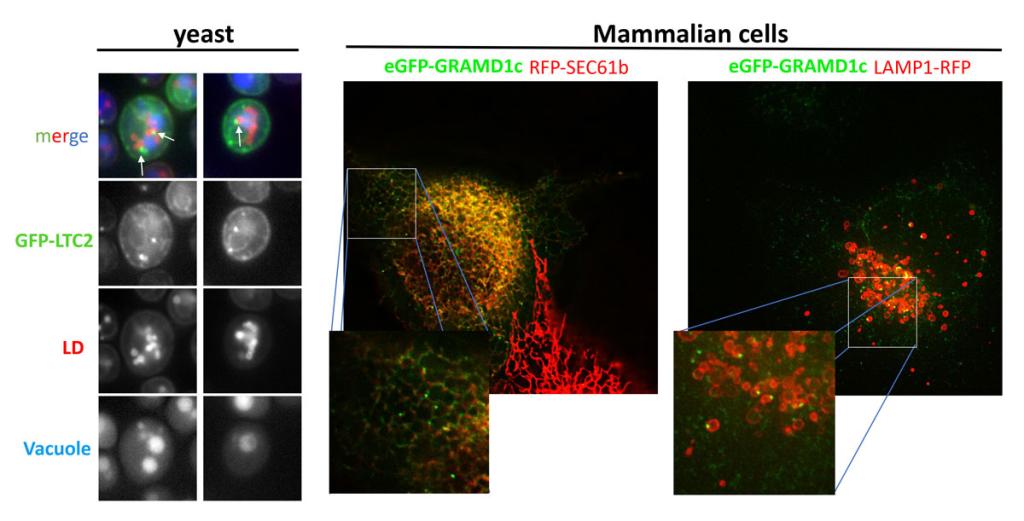
The LTP Ltc2 localizes to 3-way membrane contact sites between the ER, lipid droplets, and vacuole, the human homologue of Ltc2, GRAMD1c, have a similar localization.
Left panel, S. cerevisiae expressing GFP-Ltc2 and the LD-marker Erg6-mCherry (red) were incubated with the vacuolar dye CMAC (blue). Arrows indicate the triple junctions. Middle and right panels, COS7 cells expressing eGFP-GRAMD1c together with either the red ER marker RFP-Sec61 (middle panel) or the red lysosome marker Lamp1-RFP (right panel).
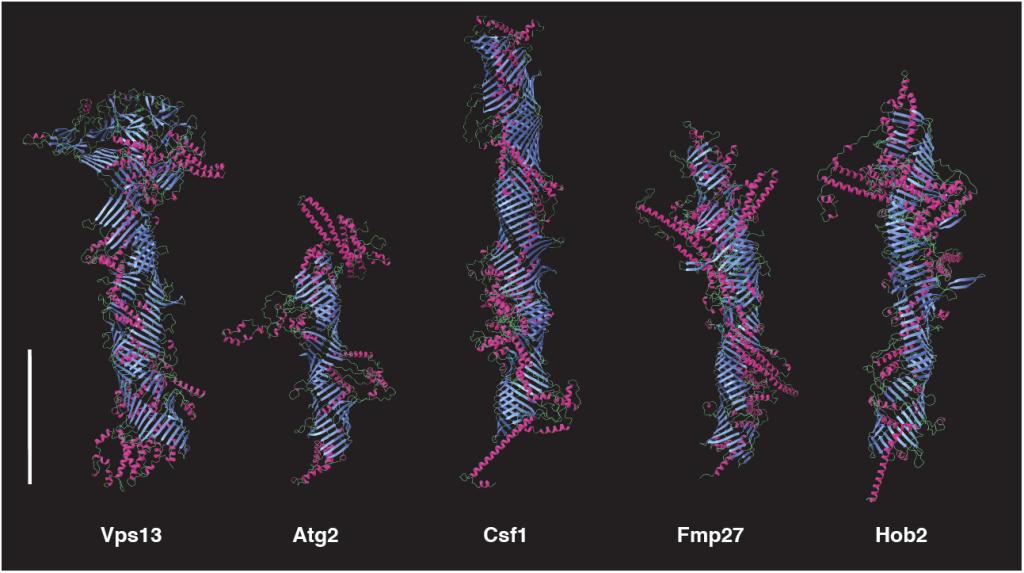
The five tube-forming lipid transport proteins in S. cerevisiae.
Structure predictions generated with RoseTTAFold: β-sheets (blue), α-helices (red), and coil (green). Bar = 10 nm.
2. Biogenesis of lipid droplets and lipoproteins in the ER.
Lipid droplets are lipid storage organelles found in all cell types. They contain a core of neutral lipids, often triglycerides and cholesteryl esters, surrounded by a monolayer of phospholipids and proteins. Nascent lipid droplets form in the ER membrane. The earliest step is thought to occur when neutral lipids in the membrane undergo a phase transition, forming lens-like structures that grow into mature lipid droplets. Accumulating evidence suggests this process occurs at specialized sites in the ER that are enriched in proteins such as seipin, LDAF1, perilipins, and MCTP2. We study early steps of LD biogenesis using model membranes and in vivo approaches in yeast and mammalian cells. Our current focus is on reconstitution of lipid droplet biogenesis in model membranes. The long-term goal is to understand what drives droplet formation and what determines the composition of lipids and proteins on the surface of lipid droplets.
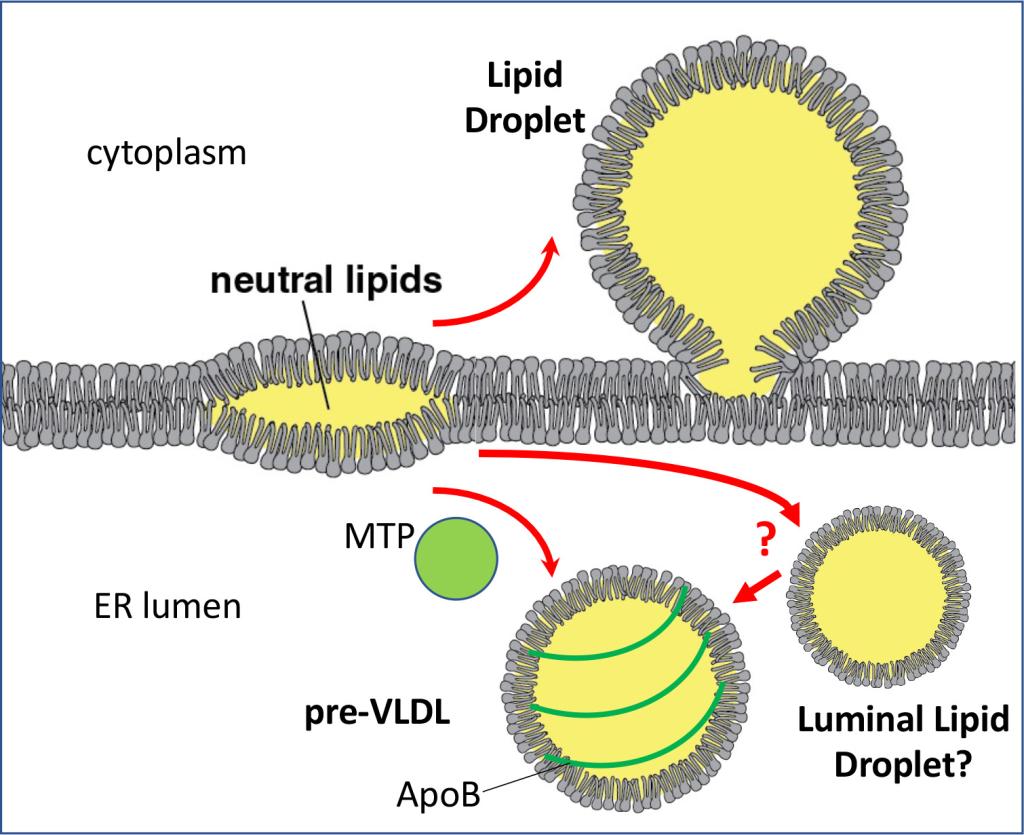
Formation of lipid droplets and lipoproteins in the ER membrane
Neutral lipids in the ER membrane undergo a phase separation and form lenses. They can grow into lipid droplets in the cytoplasm or can be used to form ApoB-coated lipoproteins in the ER lumen. Lipoprotein formation requires microsomal triglyceride transfer protein (MTP).
In vitro reconstitution of lipid droplet biogenesis on model membranes using fluorescence microscopy
Supported Lipid Bilayers (SLBs) (Left) and Giant Unilamellar Vesicles (GUVs) (Right) showing formation of lipid droplets (green) by stimulation of triacylglycerol synthesis.
Apolipoprotein B (ApoB)-containing lipoproteins are assemblies of proteins and lipids that are like lipid droplets but are secreted from cells. These particles transport dietary and endogenous lipids through the circulation. The most abundant lipoprotein in plasma is low density lipoprotein (LDL), which is metabolically derived from very low-density lipoproteins (VLDL) produced by hepatocytes in the liver. While ApoB-containing lipoproteins are essential, elevated levels in plasma contribute to prevalent metabolic diseases, including atherosclerosis, type 2 diabetes, and obesity. Developing effective therapies to safely reduce levels of these lipoproteins in plasma is a major public health goal. ApoB is required for VLDL assembly and secretion. Despite years of study, there are still major gaps in our understanding of how ApoB is lipidated and how lipoproteins are trafficked, degraded, and mature in cells. We are using high-throughput screens and proximity ligation to identify proteins required for VLDL biogenesis. The long-term goal is to understand how the lipid composition of VLDL is regulated, with an eye toward discovering drug targets.
3. How do specialized domains in the ER facilitate peroxisome biogenesis and function?
Peroxisomes play critical roles in lipid metabolism, including the β-oxidation of fatty acids and the synthesis of lipids needed for myelin generation. Defects in peroxisome formation and function cause neurodegenerative diseases such as X linked adrenoleukodystrophy and Zellweger Syndrome. More recently, it has also become clear that peroxisomes are protective organelles that play important roles in human health.
Many fundamental questions about peroxisome biogenesis remain unanswered. Cells can generate peroxisomes de novo by a process that requires production of pre-peroxisome vesicles (PPVs) in the ER. We are investigating the mechanism of PPV production and are characterizing ER subdomains where PPV production occurs. One may contain MCTP2, a reticulon-homology domain-containing proteins we found localize to subdomains in the ER that contact with both LDs and peroxisomes.
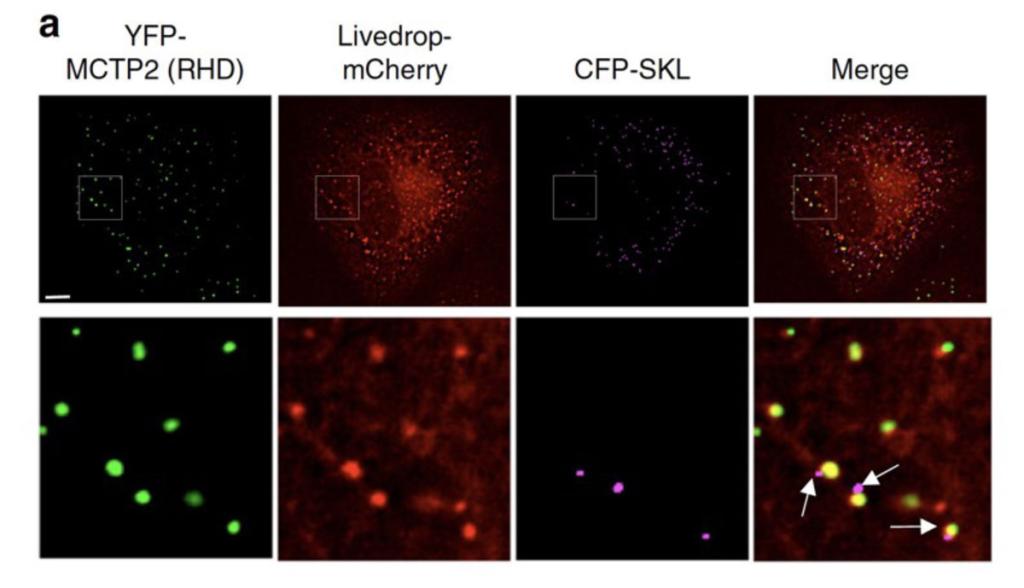
MCTP2 sites in the ER simultaneously associate with lipid droplets and peroxisomes
Images of COS7 cells transiently co-transfected with plasmids expressing YFP-MCTP2 (an ER protein enriched at sites of lipid droplet biogenesis), LiveDrop-mCherry (a lipid droplet marker), and CFP-SKL (a peroxisome marker). Arrows point to peroxisomes that are associated with lipid droplets and MTCP2 and nascent lipid droplets in the ER. Bar = 5 μm.
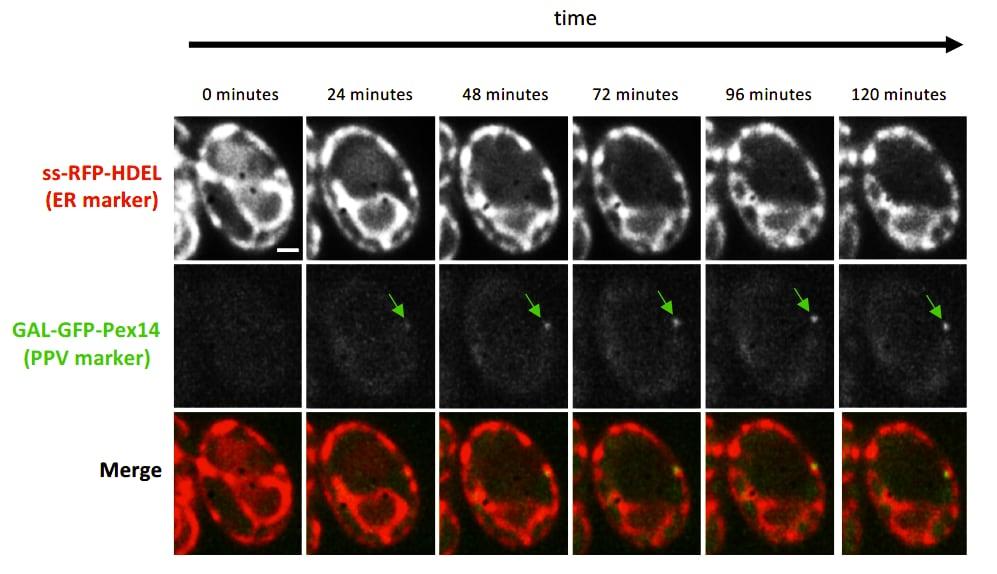
Induction of PPV formation
Time-lapse fluorescence images of pex3Δ atg1Δ cells expressing the ER marker ss-RFP-HDEL (red) and the PPV marker GFP-Pex14 (green) expressed by the galactose inducible GAL1 promoter. Time indicates number of minutes since galactose was added to the growth medium.
We are using a variety of approaches to investigate early stages of peroxisome biogenesis in mammalian cells. One is use image-based screening to identify proteins required to maintain peroxisomes in human cell lines. Machine learning and convolutional neural network models are trained to identify cells lacking peroxisomes. The models are then used to screen cells transfected with CRISPRi libraries to identify genes required to form and maintain peroxisomes.
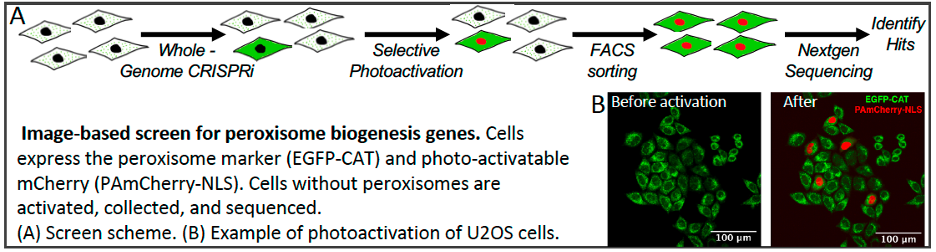