RAS Structure & Function
RAS mutations are found in ~20-30% of malignant tumors and are often essential for tumor initiation and maintenance. This makes RAS a high-priority cancer target. After decades of struggle, we now know we can target one mutant form of RAS, but many other mutants remain unaddressed. To arrive at strategies to target these, we must understand how each mutant works.
Examples of our work include:
- Systematic structural and biochemical characterization of common oncogenic KRAS mutants. This study highlighted opportunities for targeting specific RAS forms, such as the high rate of intrinsic GTPase activity of KRAS G12C that makes it susceptible to switch 2 pocket binders such as sotorasib and adagrasib.
- Identification of a major new structural class of RAS mutants including KRAS A146T and KRAS V14I that activate RAS by rapid nucleotide exchange because of switch 1 instability.
- Establishment of structural models for multimeric RAS complexes that form on cell membranes. This includes symmetric and asymmetric RAS dimer models.
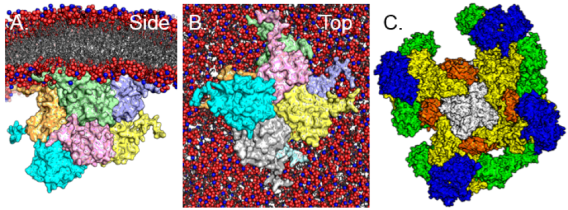
Drugging RAS
Drug hunters struggled for decades to target RAS. We and the Shokat Lab thought the G12C mutation, the most common RAS mutation in lung cancer (>20,000 cases annually in the US), would provide a foothold. Our founding laboratory project was to develop irreversible KRAS G12C inhibitors.
Examples of our work include:
- Development of SML-8-73-1, a covalent nucleotide mimetic KRAS G12C inhibitor. SML irreversibly attaches to Cysteine 12 of the oncogenic K-RAS G12C mutant and stabilizes the protein in an inactive form.
- Development of a difluoromethylene bisphosphonate analog, XY-02-082. This compound provides a foundation for development of K-RAS targeting prodrugs with improved cellular uptake.
- Development and structural characterization of the compound 4_AM. Two structurally similar KRAS G12C inhibitors, adagrasib and sotorasib, are now FDA approved.
- Demonstration that certain RAS mutants such as KRAS Q61H alter the organization of RAS signalosomes and may be amenable to targeting with RAF inhibitors.
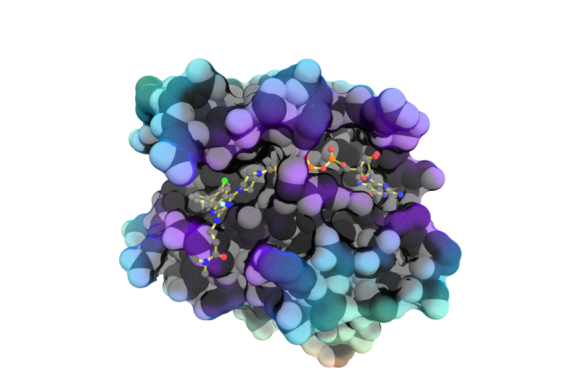
Drugging Kinases
Protein kinase inhibitors are a major class of targeted therapies, especially for cancer. Many kinases have been drugged, but there are many more to explore—both as biological tools and potential therapeutic targets. We are working to expand the arsenal of kinase inhibitors. These are often collaborative “team science” projects with our lab providing structural, biochemical, and cell biology expertise.
Examples of our work include:
- Development of DCLK1-IN-1. DCLK1-IN-1 shows exceptional selectivity for DCLK1/2 and may have a role in GI cancers.
- Development of diverse inhibitors of TAK1 including Takinib, SM1-71, and NG-25. These have potential uses in cancer, heterotopic ossification, and tissue regeneration.
- Development of the covalent SRC inhibitor 15a (aka DGY-06-116). Despite being one of the first oncogenes identified, there are no examples of targeted SRC inhibitor therapy. This tool compound may allow identification of predictive biomarkers for patient selection.
- Repurposing of ibrutinib as an inhibitor of SRMS, a regulator of autophagy through AKT signaling involved in triple negative breast cancer.
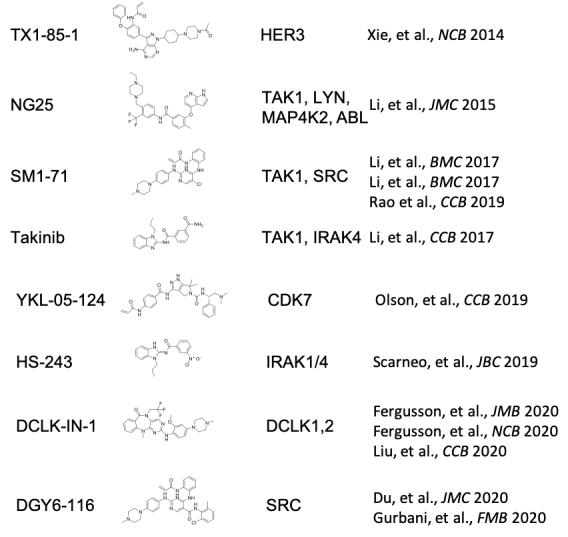
Drug Resistance
Development of drug resistance is common in cancer, especially for targeted drug therapies. Understanding drug resistance mutations is important for overcoming resistance, but can also enable biological study of potential drug targets. We are interested in using drug resistance mutations as chemical biology tools and to enable new strategies to counter drug resistance.
Examples of our work include:
- Structure-based discovery of the G532A drug resistance mutation for DCLK1. This mutation confers resistance against DCLK1-IN-1 and confirms that DCLK1-IN-1 has anti-neoplastic properties through inhibition of DCLK1.
- Structure-based discovery of the T302M drug resistance mutation for SRMS. This confers resistance to ibrutinib and validates SRMS as a therapeutic cancer target.
- Discovery of mutational profiles in KRAS G12C that confer resistance to sotorasib and adagrasib. These mutations will inform strategies to salvage patients who develop resistance and inform development of next generation drugs designed to overcome resistance.
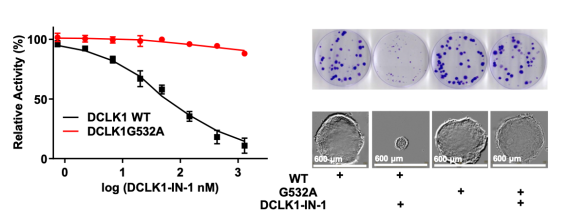
Clinical Innovation
Excellence in modern medicine requires knowledge, skill, and great effort. Computers are effort multipliers. As Director of Clinical Innovation, Dr. Westover has designed and implemented multiple technologies that are in clinical use today that improve the efficiency and quality of care in radiation oncology.
Examples of work in this area include:
- A clinic room utilization system called RO-PAD
- Leveraging direct 3D printing to make custom 3D boluses for complex radiation treatments
- Automated reminders to patients to improve compliance with appointments
- Wait time displays for patients waiting for radiation treatments
- Tools in the EPIC electronic medical record system to improve communication and documentation
- A system for export of graphical radiation plans to PACS where all providers can see them
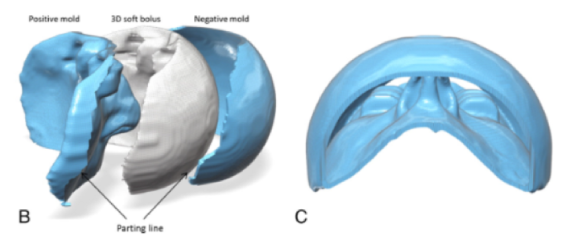
Clinical Research
Dr. Westover is a practicing thoracic radiation oncologist with additional interests in cancers in the central nervous system.
Examples of work in this area include:
- Clinical trials in proton SBRT for lung cancer
- Clinical trials in hypofractionated radiation for patients with locally advanced non-small cell lung cancer
- Clinical trials of SBRT in the oligometastatic setting
- Clinical trials of hippocampal-sparing whole brain radiation.
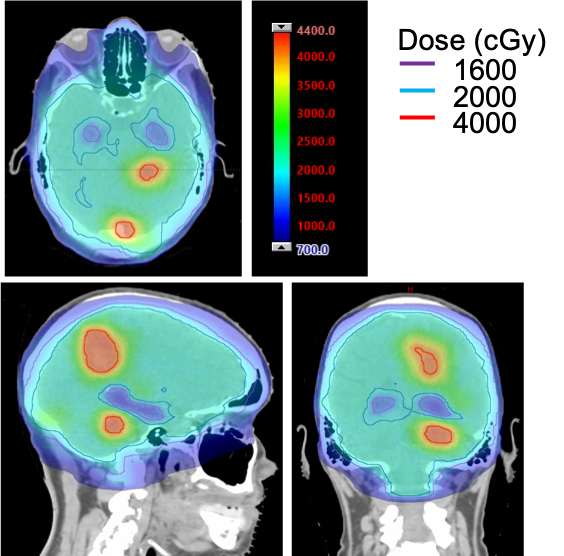