Research Overview
Our long-term goal is to elucidate host-pathogen interactions that govern the ability of Mycobacterium tuberculosis (Mtb) to cause disease in humans. We are taking an integrated approach, using powerful new molecular genetic, cell biologic, bioinformatic, and metabolomic tools to test novel hypotheses and challenge existing paradigms. Our ultimate aim is to use this knowledge to develop new vaccines and treatments for Mtb (Fig. 1).
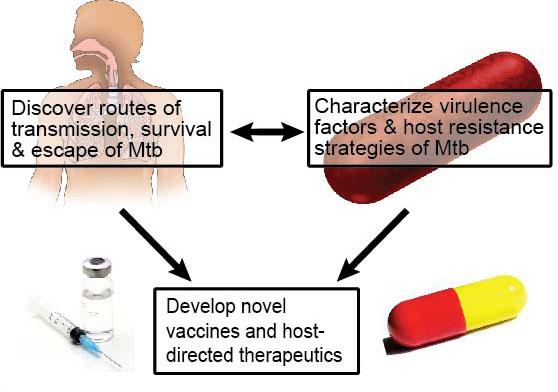
Figure 1: An integrated approach towards prevention & cure of Mtb. We combine the study of host-pathogen interactions with genetic and biochemical analyses to discover novel biology and therapeutic directions.
Discovering the routes of transmission, survival and escape of Mtb from its human host
Three key phases in a pathogen’s life cycle dictate its ability to cause disease, namely, i) invasion ii) survival and propagation and iii) escape beyond the host to infect naïve individuals. To date, how Mtb crosses the mucosa and enters the human body is incompletely understood. Likewise, the full repertoire of mechanisms used by Mtb to manipulate and persist within host macrophages is unknown. Additionally, our knowledge of macrophage antimicrobial mechanisms in host defense against Mtb and other pathogens remains incomplete. Enhancing such antimicrobial mechanisms via host-directed therapies is a promising new approach to Mtb treatment. Finally, how Mtb facilitates its own transmission through cough induction has not been studied. Thus, we are addressing these areas of Mtb biology through a series of hypothesis-driven approaches. First, how does Mtb penetrate the nasopharyngeal and respiratory mucosa to cause disease? Second, what are the mechanisms Mtb uses to manipulate host processes to facilitate survival? Third, can host antimicrobial pathways be leveraged to enhance the eradication of intracellular bacteria such as Mtb? Finally, how does Mtb trigger coughing to mediate its spread?
Research Questions
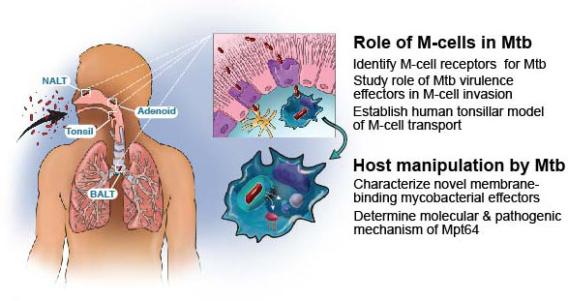
Figure 2: Schematic of the initial entry, survival and propagation of Mtb in macrophages. Aerosolized Mtb interact with MALT (NALT, tonsils, adenoids, and BALT) where they contact and are translocated by microfold cells (purple, upper panel). Macrophages either in alveoli or in the submucosa below M-cells ingest Mtb, and Mtb secretes membrane binding virulence factors or small molecules to modulate the host immune response (lower panel).
How does Mtb cross the airway mucosa?
To address the question of how Mtb crosses the airway mucosa to establish infection, we are testing the hypothesis that a rare epithelial cell called a microfold or M-cell mediates Mtb transmission (Fig. 2).
In the oropharynx and respiratory tree, Mtb encounters mucosa associated lymphoid tissue (MALT) such as nasal associated lymphatic tissue (NALT), tonsils, and adenoids. Specialized M-cells overlying MALT transcytose antigens from the apical to the basolateral compartment. Whether Mtb leverage M-cells to initiate infection is unknown. We recently established a mouse intranasal infection model and found that cervical lymph node dissemination by Mtb occurred without contacting alveolar macrophages. Mice genetically lacking M-cells or depleted of M-cells had reduced Mtb invasion and dissemination to draining lymph nodes. M-cell depletion delayed mouse mortality in a low-dose aerosol infection, indicating a vital role for M-cells in respiratory Mtb disease.
Our ongoing experiments are aimed at identifying how Mtb adheres and traffics through M-cells, and the consequences of M-cell transcytosis for innate and adaptive immunity. We have preliminary data that a cell surface receptor on M-cells mediates transcytosis, and are characterizing this interaction with Mtb in vitro and in vivo. We are also determining how M-cell transcytosis influences the subsequent interaction of Mtb with macrophages and dendritic cells. Finally, since M-cells overlie human tonsils, we are establishing an M-cell translocation assay in human tonsillar explants to assess the role of M-cells, Mtb receptors, and transcytosis in primary human tissue. The identified mechanisms will fundamentally advance the fields of microbiology and host defense, and provide new approaches towards reducing Mtb infectivity.
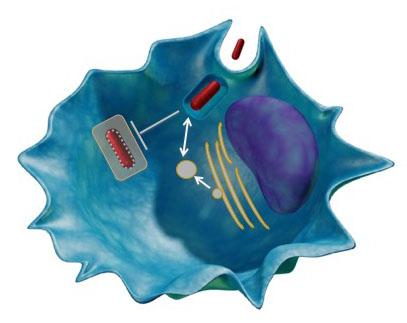
Figure 3: Identification of Mtb exported proteins. Model showing examples of Mtb virulence mechanisms including promoting cell entry, arresting phagosome maturation, and interaction with host secretion machinery.
How does Mtb manipulate the host to facilitate survival?
A growing body of evidence indicates that Mtb actively modulates host membrane dynamics by arresting phagosome maturation, preventing phagosomal acidification, and inhibiting autophagy. How Mtb achieves these outcomes remains unknown. Because i) many intracellular pathogens secrete virulence factors that modulate host membrane dynamics, ii) Mtb secretes ~10% of its proteome and iii) Mtb actively engages multiple membrane-dependent host processes, we hypothesized that some secreted mycobacterial proteins might interact with host membranes to influence the outcome of infection (Fig. 2, 3). We employed a novel high-throughput live-dead screen in Saccharomyces cerevisiae to screen two hundred putative Mtb effectors for membrane binding and identified ~50 membrane binding effectors. We are have extensively characterized one effector, Mpt64, a highly secreted and immunogenic protein of unknown function, and are now further characterizing multiple other effectors for their virulence activities.
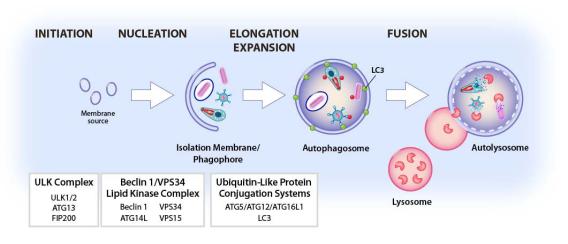
Can autophagy be harnessed to enhance the killing of Mtb?
Autophagy is an evolutionarily conserved cellular pathway in which cytoplasmic constituents including organelles and microbes or microbial constituents called “cargo” are enveloped in a double membrane-bound vesicle, the autophagosome, which fuses to the lysosome to create the autolysosome. Cargo is then degraded by lysosomal enzymes. All eukaryotic cells perform autophagy, which plays a key role in a range of cellular and physiologic processes including resistance to infection and regulation of immunity. Autophagy can capture either whole pathogens or pathogen components as cargo, resulting in killing of pathogens or clearance of pathogen-encoded molecules (Fig. 4).
Degradative autophagy can target intracellular pathogens, including Mtb, to the lysosome for destruction. While Mtb can block autophagy, we have shown that degradative autophagy can be induced to control Mtb infection and have identified mechanisms involved in triggering degradative autophagy during Mtb infection. We identified key roles for the cytoplasmic DNA sensor cGAS and its downstream signaling partner Sting in autophagy initiation and roles for the ubiquitin ligases Smurf1 and Parkin in targeting Mtb for degradation. An important validation of our approach to increase degradative autophagy and its physiologic importance is that autophagy-enhancing compounds reduce Mtb replication. For example, a peptide developed to enhance autophagy also enhances killing of Mtb.
On the basis of our previous work, we hypothesized that molecules that enhance autophagy could be advanced as host-directed therapies for Mtb infection. To that end, in a collaboration with labs at UTSW, Washington University of St. Louis, Harvard/Broad Institute, Scripps Institute and Vir pharmaceuticals, we are identifying new targets for enhancing autophagy, and developing established molecules from high-throughput screens. We anticipate that this work will lead to novel therapeutic approaches towards the treatment of Mtb and other important pathogens.
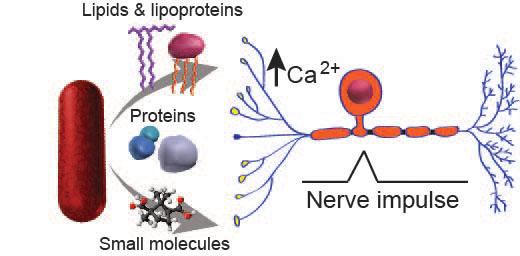
How does Mtb mediate cough and transmission?
The success of any bacterial pathogen ultimately depends on its ability to multiply and infect new hosts. Many pathogenic bacteria interact with hosts by secreting virulence molecules like proteins and lipids. A classic example is the production of cholera toxin by Vibrio cholera, which enhances the pathogen’s spread from person-to-person by promoting profuse watery diarrhea. Mtb, one of the most successful pathogens in human history, also employs sophisticated means of dissemination, including mediating caseation, tissue destruction, and airborne transmission, to propagate the human disease tuberculosis. Yet, despite the massive toll of Mtb on world health, the molecular mechanisms responsible for Mtb transmission remain elusive. Cough is a primary symptom of active pulmonary tuberculosis and is known to be a critical mechanism of transmission. Although cough is a major route of aerosolization and spread of Mtb, very little is known about the molecular factors that produce cough during human infection. Thus, there is an urgent need to identify bacterial factors that induce cough. Because airway nociceptive neurons can trigger cough and some bacteria, including mycobacteria, secrete complex molecules targeting neurons, we hypothesized that Mtb secretes molecules triggering nociceptive neurons to activate the cough response thereby facilitating disease transmission.