The vertebrate Shh pathway, primary cilium, and GPCR signaling
The vertebrate Shh pathway is one of the best examples where the primary cilium has been implicated in cellular signaling (Goetz and Anderson, 2010). The final output of the Shh pathway is the formation of Gli transcriptional repressors or activators, both of which occur in a cilia-dependent manner. While the Gli3 repressor is critical in basal suppression of the pathway, the Gli2 activator is the major activator for signaling. The Gli2 transcriptional activator is formed by a Smo-dependent process, which is initiated upon binding of Shh to Ptch1, removal of Ptch1 from cilia (Rohatgi et al., 2007), and ciliary retention of Smo (Corbit et al., 2005). The Gli3 repressor is formed in a protein-kinase A (PKA)-dependent manner by limited proteolysis, with the N-terminus acting as a transcriptional repressor (Mukhopadhyay and Rohatgi, 2014; Tuson et al., 2011). Phenotypes resulting from loss of cilia depend on the predominance of the role of repressor or activator in development and patterning of the particular tissue. For example, disruption of cilia in the neural tube results in decreased Shh signaling, predominantly in a Gli2-activator dependent manner (Goetz and Anderson, 2010).
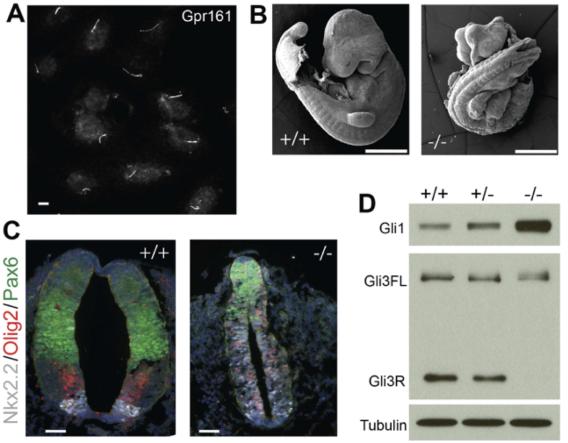
Loss of Tulp3 and IFT-A complex results in increased Shh signaling in the neural tube, as opposed to decreased signaling with loss of cilia (Norman et al., 2009; Ocbina et al., 2011; Qin et al., 2011). This suggests that there are Tulp3/IFT-A-regulated negative regulators of Shh signaling. We discovered that the orphan GPCR, Gpr161 localizes to cilia in a Tulp3/IFT-A-regulated manner and negatively regulates Shh signaling via cAMP signaling (Mukhopadhyay et al., 2013). A null murine allele of Gpr161 phenocopies Tulp3/IFT-A mutants in causing increased Shh signaling with concomitant lack of Gli3 repressor. Constitutive cAMP signaling by Gpr161 suggest that it regulates PKA-mediated Gli repressor formation, possibly by increasing cAMP levels in cilia (Mukhopadhyay et al., 2013). As cAMP production by GPCRs is mediated by Gs coupling and activation of adenylyl cyclases, it is interesting to note that Gnas (Gs) knockout mice exhibit increased Shh signaling (Regard et al., 2013). Finally, cAMP binds to PKA regulatory subunits, which are spatially restricted by A-kinase anchoring proteins (AKAPs), promoting release of PKA catalytic subunits in close vicinity. PKA catalytic subunit mutants demonstrate increased Shh signaling (Tuson et al., 2011). Interestingly, PKA regulatory subunits that localize to cilia (Mick et al., 2015) directly bind to an amphipathic helix at the Gpr161 distal C-terminal tail (Bachmann et al., 2016) suggesting that Gpr161-PKA coupling occurs in cilia, with Gpr161 functioning as an AKAP. Key future directions here are to determine if lack of Gpr161 localization to cilia results in a phenotype similar to lack of Gpr161. In addition, phenotypic characterization of Gpr161 conditional mutants should provide important clues regarding the role of this GPCR in basal suppression of hedgehog pathway in normal development and in pathogenesis of Shh-dependent tumors.
References
Bachmann, V.A., Mayrhofer, J.E., Ilouz, R., Tschaikner, P., Raffeiner, P., Rock, R., Courcelles, M., Apelt, F., Lu, T.W., Baillie, G.S., et al. (2016).
Gpr161 anchoring of PKA consolidates GPCR and cAMP signaling. Proceedings of the National Academy of Sciences of the United States of America 113, 7786-7791.
Corbit, K.C., Aanstad, P., Singla, V., Norman, A.R., Stainier, D.Y., and Reiter, J.F. (2005). Vertebrate Smoothened functions at the primary cilium. Nature 437, 1018-1021.
Goetz, S.C., and Anderson, K.V. (2010). The primary cilium: a signalling centre during vertebrate development. Nat Rev Genet 11, 331-344.
Mick, D.U., Rodrigues, R.B., Leib, R.D., Adams, C.M., Chien, A.S., Gygi, S.P., and Nachury, M.V. (2015). Proteomics of Primary Cilia by Proximity Labeling. Developmental cell 35, 497-512.
Mukhopadhyay, S., and Rohatgi, R. (2014). G-protein-coupled receptors, Hedgehog signaling and primary cilia. Semin Cell Dev Biol 33, 63-72.
Mukhopadhyay, S., Wen, X., Ratti, N., Loktev, A., Rangell, L., Scales, S.J., and Jackson, P.K. (2013). The ciliary G-protein-coupled receptor Gpr161 negatively regulates the Sonic hedgehog pathway via cAMP signaling. Cell 152, 210-223.
Norman, R.X., Ko, H.W., Huang, V., Eun, C.M., Abler, L.L., Zhang, Z., Sun, X., and Eggenschwiler, J.T. (2009). Tubby-like protein 3 (TULP3) regulates patterning in the mouse embryo through inhibition of Hedgehog signaling. Hum Mol Genet 18, 1740-1754.
Ocbina, P.J., Eggenschwiler, J.T., Moskowitz, I., and Anderson, K.V. (2011). Complex interactions between genes controlling trafficking in primary cilia. Nat Genet 43, 547-553.
Qin, J., Lin, Y., Norman, R.X., Ko, H.W., and Eggenschwiler, J.T. (2011). Intraflagellar transport protein 122 antagonizes Sonic Hedgehog signaling and controls ciliary localization of pathway components. Proc Natl Acad Sci U S A 108, 1456-1461.
Regard, J.B., Malhotra, D., Gvozdenovic-Jeremic, J., Josey, M., Chen, M., Weinstein, L.S., Lu, J., Shore, E.M., Kaplan, F.S., and Yang, Y. (2013). Activation of Hedgehog signaling by loss of GNAS causes heterotopic ossification. Nat Med 19, 1505-1512.
Rohatgi, R., Milenkovic, L., and Scott, M.P. (2007). Patched1 regulates hedgehog signaling at the primary cilium. Science 317, 372-376.
Tuson, M., He, M., and Anderson, K.V. (2011). Protein kinase A acts at the basal body of the primary cilium to prevent Gli2 activation and ventralization of the mouse neural tube. Development 138, 4921-4930.